History and current pharmacological applications of Ketamine: from an analgesic to an anti-depressive drug
The history of ketamine: from anaesthesia to treatment-resistant depression
The journey of ketamine began in the 1950s as Parke-Davis and Company in Detroit sought to create a potent anaesthetic and were exploring cyclohexylamines to this end. Six years later, V. H. Maddox developed a process that led to the synthesis of phencyclidine or PCP [N-(1-phenyl-cyclohexyl)-piperidine]. On 11 September 1958, Parke-Davis began studying the experimental effects of the drug on animals. They found that PCP created powerful analgesia in animals and allowed monkeys to undergo surgical incisions without pain. However, the animals entered a cataleptic state with their eyes open, and the quality of muscle relaxation was poor.

Figure 1. A graphic history of the discovery and development of ketamine
Later in the same year, Parke-Davis conducted research on the potential of PCP as a human anaesthetic under the trade name of Sernyl. The first human trials of PCP in 1958 showed that PCP produced genuine narcosis, a cataleptic state, potent amnesia, and analgesia. Researchers concluded that phencyclidine produced a "centrally mediated" sensory deprivation syndrome. While some surgical incisions and complete operations could be performed using PCP alone, it was unsatisfactory for surgery in some patients due to severe excitation. Other related compounds including eticyclidine (CI-400 or PCE) were trialed but were found to produce unwanted hallucinations.
Undeterred, the researchers continued their quest and synthesized a new compound called ketamine. In 1963, it was patented and initially used as a veterinary anaesthetic. However, its true potential was yet to be unveiled.
One year later, the first human administration was conducted to volunteer prisoners at the Jackson Prison in the state of Michigan (yes, this sentence can give you goosebumps). Patients described feelings of floating in outer space and having no feeling in their limbs. The study, published in 1965, highlighted the cataleptic, analgesic, and anaesthetic effects, and the surprising lack of hypnotic properties. Ketamine became available by prescription in 1969 in the form of ketamine hydrochloride, named Ketalar. It was officially approved for human consumption by the United States Food and Drug Administration in 1970 and, because of its sympathomimetic properties and its wide margin of safety, was administered as a field anaesthetic to (and abused by) soldiers during the Vietnam War. Ketamine is still used today as anaesthetic due to the main advantages that it is fast-acting and can provide rapid anaesthesia with minimal respiratory depression, which makes it particularly useful in emergencies requiring rapid onset anaesthesia.
In 1980, a groundbreaking discovery revealed that ketamine is a noncompetitive inhibitor of N-methyl-D-aspartate calcium channels (NMDA) receptors in the brain. This revelation opened doors to understanding its effects on mental health. NMDA is responsible for the induction of synaptic plasticity, and NMDA receptor blockade made rodents unable to form spatial memories. The discovery of the NMDA receptor and its noncompetitive inhibition by ketamine prompted large advances in the pathophysiology of hyperalgesia, schizophrenia and mental functioning. At the same time, researchers started to explore the possible connection between depression and dysfunction in the glutamatergic system.
Glutamate: Not Always Your Friend
Dysfunction in the glutamatergic system refers to abnormalities or imbalances in the function of neurotransmitters and receptors that use glutamate, which is the most abundant excitatory neurotransmitter in the brain. This dysfunction may involve alterations in the basal level, release, or uptake of glutamate, as well as changes in the function or expression of the receptors that respond to it, including NMDA receptors.
Trullas and Skolnick were among the first researchers to explore depression and glutamatergic dysfunction. They observed that NMDA receptor activation could cause behavioural symptoms of depression and impair long-term potentiation in the hippocampus. Their preclinical studies demonstrated that the blockade of NMDAR with ketamine had significant antidepressant properties comparable to conventional antidepressants. Despite this promising preclinical evidence, it was not until the early 2000s that ketamine was tested as an antidepressant agent in human subjects. Since then, numerous placebo-controlled studies have shown that subanesthetic-dose ketamine can exert rapid, robust and relatively sustained antidepressant effects on some patients.
However, researchers realized that the side effects of ketamine could limit its use as an antidepressant. To address this issue, they began to investigate the different enantiomers of ketamine, which are mirror images of each other and can have different pharmacological properties. They found that one enantiomer, (S)-ketamine or esketamine, had fewer dissociative side effects than the racemic mixture of ketamine, while still retaining its antidepressant properties.
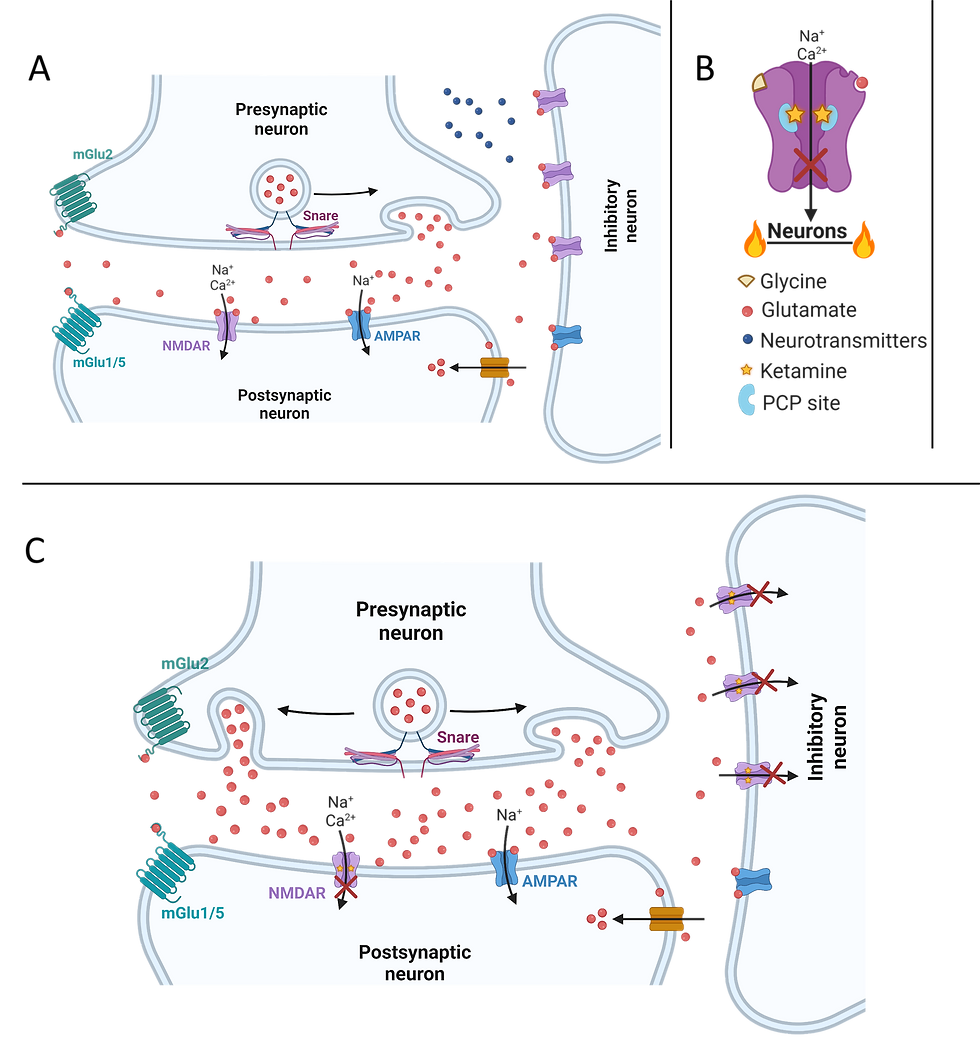
Figure 2. Graphics showing the mechanism of ketaminergic action at the synapse and receptor level. A) Baseline interaction in a synapse with an inhibitory GABAergic interneuron inputting onto the synaptic connection between two excitatory neurons. The inhibitory neuron releases neurotransmitters which regulate glutamatergic communication in the synapse. B) Blockade of the calcium channel pore in response to ketamine binding to the PCP site in a singular NMDAR, preventing neuronal activation. C) The synapse from Figure A after administration of ketamine. Inhibitory action from the GABAergic interneuron is decreased during NMDAR blockade, and glutamatergic transmission between the two excitatory neurons consequently increases.
Before digging deeper into the pharmacological differences between (S)-ketamine and (R)-ketamine as antidepressant drugs, is important to better understand depression and the current methods of clinical assessment when evaluating a potential antidepressant drug.
Depression: what is it in a nutshell
Depression is a heterogeneous psychiatric disorder that affects mood, behaviour and overall health. The broad range of symptoms is not present in every patient, making it difficult to treat every patient with a single drug. Symptoms include anhedonia, changes to appetite and sleeping patterns, loss of energy, and difficulty concentrating. Physical symptoms are also common, ranging from unexplained aches and pain, to gastrointestinal problems, tiredness and psychomotor activity changes.
Medication has been given for depression for well over a century – from lithium in the late 1800’s, to monamine-modulating drugs after they were accidentally discovered to have antidepressant properties during the treatment of allergies and tuberculosis (imipramine and iproniazid, respectively) in the 1950’s.
Despite this long history, even modern pharmacological solutions leave much to be desired. Firstly, antidepressants can take up to three weeks before reaching peak effectiveness, during which suicidality is reportedly increased for some individuals. Secondly, a wide range of side effects are reported including sexual dysfunction, weight gain, emotional blunting, nausea, dry mouth and insomnia. Finally, between 20-40% of patients are classified as Treatment Resistant i.e. do not respond to antidepressant drugs, and some 60-70% of all patients do not experience a full remission of symptoms.
It’s no wonder then, that the hunt is on for a faster-acting, more efficacious drug with fewer side effects. However, there are several difficulties in researching a new compound. Besides the heterogeneous nature of the disorder and the degrees of patient’s treatment resistance, there is also the question of how to measure depression pre-clinically. The usual methods of clinical assessment – clinician administered or self-reported questionnaires such as the Hamilton Depression Rating Scale – do not cut the mustard when working with rodent models – you cannot ask a rat how they are feeling today. There is still debate as to the validity of the tests used instead: the Forced Swim and Tail Suspension tests both involve placing a rodent in an unpleasant situation and timing how long it takes for them to give up trying to escape; while the sucrose preference test appears to effectively measure anhedonia but not other depressive symptoms.
Pharmacological and biochemical differences between (R)-ketamine and (S)-ketamine
Ketamine was discovered and is usually administered as a racemic mixture, (R/S)-ketamine. In the past 20 years, anesthesiologists have preferred the use of the more potent (S)-enantiomer (Ketanest®) as it grants greater control over and recovery from anaesthesia.
In the context of Treatment-Resistant Depression (TRD) results have been difficult to interpret. Positive response rates in TRD patients of 67% or 91% have been seen after infusions of (S)-ketamine (0.4 and 0.2 mg/kg) or (R/S)-ketamine (0.5 mg/kg) respectively, but what constitutes a “positive response” varies between studies.
In 2019, an intranasal preparation of (S)-ketamine was developed and received approval from the US FDA and EMA for the treatment of TRD. However, the approval came with restrictions due to a few reasons. Firstly, out of the five studies conducted for approval, only two showed positive results. Secondly, there were concerns about serious adverse effects associated with the use of (S)-ketamine. To address these risks, the approval was granted under the Risk Evaluation and Mitigation Strategy.
In humans, a recent open-label study with (R)-ketamine showed antidepressant efficacy without dissociative effects. However, a clinical-stage biopharmaceutical company focused on mental health announced that while R-ketamine (PCN-101) demonstrated signals of efficacy up to two weeks, the Phase 2a clinical trial did not meet its primary endpoint: a statistically significant change from baseline in the participants’ MADRS (Montgomery-Åsberg Depression Rating Scale) score at 24 hours compared to placebo.
Ketamine, widely recognized as a non-competitive antagonist of the N-methyl-D-aspartate receptor (NMDAR), exerts its primary influence by binding to the allosteric phencyclidine (PCP) site within NMDAR. Despite being the primary target, ketamine's interaction with NMDARs occurs within a narrow range of affinity in the micromolar scale, demonstrating limited selectivity for these receptors in comparison to other recognized or unidentified receptors and cellular targets.
How does the inhibition of NMDAR influence the glutamate signalling?
Within the brain, excitatory neurons elicit firing from neurons, while inhibitory neurons (primarily GABAergic interneurons) tightly regulate excitatory neurons. It is believed that ketamine hinders the function of NMDA receptors on the inhibitory neurons responsible for the suppression of neuronal activity. According to Sanacora, a researcher at Yale, ketamine selectively obstructs the NMDA receptor on inhibitory neurons, thereby augmenting their activation (figure 2). In essence, ketamine impedes the inhibitory neurons' ability to restrain neuronal activity, resulting in an amplified glutamate signalling.
The concept of suppressing inhibitory neurons has prompted scientists to explore the antidepressant potential of other NMDA receptor blockers and antagonists. However, clinical trials involving blockers such as memantine, commonly used for dementia treatment, and AstraZeneca's lanicemine, have yielded inconsistent outcomes.
In light of ketamine's history of being abused, the potential involvement of the brain's opioid systems, which are closely associated with substance abuse, intrigued Schatzberg’s research team. In 2018, Schatzberg and his colleagues discovered that administering naltrexone to individuals before ketamine administration prevented the antidepressant effect of ketamine. Naltrexone is commonly prescribed to aid individuals dependent on opioids in their withdrawal process. Its mechanism of action involves blocking the mu-opioid receptor in the brain, which is activated by morphine and similar substances.
Interestingly, both enantiomers of ketamine possess an equal affinity for mu-opioid receptors (MORs), but it is noteworthy that (S)-ketamine exhibits around six times greater affinity for NMDARs in mice (Ki = 0.8 μM) when contrasted with (R)-ketamine (Ki: 5 μM).
Recently, Bonaventura et al. conducted a study to explore potential off-target effects of both R and S ketamine using fluorescent probes and rat models. Their findings suggest that neither R nor S ketamine bind strongly to specific targets or exhibit significant off-target binding. In other words, they do not strongly bind to receptors or enzymes beyond the ones already identified. The study also revealed that the uptake of ketamine in the brain is rapid, likely due to the compounds' low affinity for any biological target in the brain. Essentially, ketamine easily passes through the blood-brain barrier but does not strongly bind to receptors or enzymes and is thus released back into the bloodstream.
Despite being weak binders in general, low doses of intravenous (S) or (R) -ketamine show different effects on metabolic activity and dopamine levels in various regions of the brain. Notably, (S)-ketamine increases metabolic activity, dopamine levels, and opioid receptor signalling. Furthermore, exposure to subanaesthetic doses of (S) ketamine in mice results in significantly higher rates of locomotor activity and self-administration of the drug compared to (R)-ketamine.
Remarkably, the effects of (S)-ketamine on locomotion are partially blocked by naltrexone, an opioid antagonist commonly used to treat alcohol use disorder and opioid dependence. Based on the pharmacological profile of (S)-ketamine and the mice’s increased self-administration, researchers speculated that it could have a higher potential for abuse compared with (R)-ketamine. However, the complex pharmacological profile of ketamine and its relationship to therapeutic effects and abuse liability is still not fully understood. Additionally, mice showed psychomotor sensitization to repeated injections of (S)-ketamine but not (R)-ketamine, similar to the effects observed with opioid and psychostimulant drugs.
Therefore, the authors of the study concluded that the primary reason for the abuse liability of racemic ketamine in humans lies in the pharmacological effects of its (S)-enantiomer. However, other specialists argue that if the (R)-ketamine were to be utilized for depression treatment, achieving an antidepressant effect would likely necessitate an increase in dosage to attain equipotency for NMDAR antagonism. This would be true even if stimulation of mu-opioid receptors (MORs) played a role in the antidepressant mechanism. At such elevated doses, it is anticipated that (R)-ketamine would exhibit comparable abuse liability. Therefore, they argue that the study designs and results do not sufficiently support this claim. Consequently, they caution against drawing translational interpretations from these data without considering the inherent limitations of the study designs.
Catabolize to Therapize: Metabolites of Ketamine
Interestingly, a Nature article from 2016 revealed the significance of the metabolism of (R/S)-ketamine into (2S, 6S; 2R, 6R)-hydroxynorketamine (HNK) for its antidepressant effects. In mice, the (2R, 6R)-HNK enantiomer demonstrated various antidepressant-related actions at the behavioural, electroencephalographic, electrophysiological, and cellular levels. These metabolites act as weak inhibitors of NMDAR, with (2S, 6S)-HNK and (2R, 6R)-HNK exhibiting Ki values of 21.19 μM and > 100 μM, respectively. However, they are more potent and selective negative allosteric modulators of the α7-nicotinic acetylcholine receptor, with an IC50 below 1 μM. Notably, (2R,6R)-HNK does not produce side effects typically associated with ketamine. Although the mechanism of action of HNK remains unclear, it is presumed that instead of blocking the NMDA receptor, (2R,6R)-HNK enhances the activation of the AMPA receptor through an unknown mechanism.
It is worth mentioning that (2R,6R)-HNK is currently being developed by the National Institute of Mental Health (NIMH) in the United States for the treatment of depression. The results from a double-blind phase I clinical trial involving 74 healthy volunteers are expected to be disclosed very soon (https://clinicaltrials.gov/ct2/show/NCT04711005).
Promising Uses of (S)-ketamine: Not Just a Treatment for Drug-Resistant Depression
(S)-Ketamine is not being tested just for TRD but also for other mental disorders:
For substance use disorders (SUDs), a randomized controlled trial found that a single dose of intranasal (S)-ketamine was associated with decreased craving and drug use in individuals with cocaine use disorder. Another study suggested that repeated intranasal (S)-ketamine treatment may improve symptoms of alcohol use disorder, although further research is needed.
For post-traumatic stress disorder (PTSD), a review of clinical trials found that (S)-ketamine nasal spray may improve PTSD symptoms, although more research is needed to determine optimal dosing and long-term effects.
One recent article highlighted the potential of ketamine to rapidly reduce PTSD symptoms, potentially through its effects on glutamate and brain-derived neurotrophic factor (BDNF) pathways. The authors also discussed ongoing research on the use of ketamine in combination with other therapies, such as cognitive-behavioural therapy, for the treatment of PTSD.
For bipolar depression, a systematic review and meta-analysis suggested that intranasal (S)-ketamine may be effective in reducing depressive symptoms in individuals with bipolar disorder.
Finally, for eating disorders, a case report described a patient with severe anorexia nervosa who experienced significant improvement in symptoms following treatment with intranasal (S)-ketamine.
Overall, while there is some promising evidence for the use of (S)-ketamine in these disorders, further research is needed to determine optimal dosing, long-term effects, and potential risks associated with its use.
Rounding off
As the hunt for a faster-acting, more effective antidepressant continues, ketamine's journey from an anaesthetic to a potential treatment for treatment resistant depression amongst other disorders is a testament to the power of scientific discovery. While there are still concerns about its abuse potential, and more research is needed to elucidate why it is more effective in some patients than others, it has provided new avenues of hope for those battling depression.
Interesting articles
Main review for the history of ketamine
Review article on novel drug developmental strategies for treatment-resistant depression
The discussed article by Bonaventura et Al.
and the related comment
In case you want to dig into the mechanisms of ketamine and its metabolites as antidepressants
Lorenzo Cianni and Christien Bowman (the editor of this article)